A Mechanism Reproducing Jaw Biomechanics

Purchased on Istockphoto.com. Copyright.
Although studies have shown that jaw movements deform the earcanal, their interactions are not fully understood. The objective of this study is to design a jaw simulator that, combined with an artificial ear, will make it possible to study the anatomical coupling of the jaw and earcanal. The proposed algorithm is intended for the design of a mechanism that reproduces jaw kinematics with an accuracy of 98.35%. A better understanding of the relationship between jaw and earcanal would help improve earplug ergonomics and especially the comfort and acoustic sealing of in-ear technologies. Keywords: temporomandibular joint, biomechanics, mechanical design
Jaw and Earcanal: An Anatomical Relationship that Remains Misunderstood
Surprising as it may seem, movements of the temporomandibular joint (TMJ), better known as the jaw joint, affect the shape of our earcanal [1]. This phenomenon, which researchers refer to as a “dynamic earcanal movement,” has attracted considerable interest. Indeed, studies have been conducted to evaluate the energy potential of earcanal deformations induced by jaw movements [2, 3]. Although the results are promising, studies have only focused on the earcanal and not on modeling the behaviour of the jaw. This is due to a lack of knowledge of the anatomical relationship between earcanal and jaw. This lack of knowledge hinders the precise characterization of this energy source’s potential.
To address the issue, the objective of our study is to design a mechanism that reproduces jaw biomechanics in order to study the anatomical relationship of the earcanal and the TMJ.
Biomechanics of the Temporomandibular Joint (TMJ)
The TMJ is a double joint connecting the jaw to the skull through mandibular condyles. Each mandibular condyle is located in a part of the skull called the temporal fossa. The mandibular condyle moves along the temporal fossa profile when the mouth is opening. The virtual axis passing through the centre of the two mandibular condyles, called “hinge axis”, is frequently used as a reference in biomechanics. It is from this axis, as shown in Figure 1, that the three parameters describing the kinematics of jaw openings are defined: (1) the condylar path, the path taken by the centre of the mandibular condyle [4]; (2) the incisal path, the path taken by the tip of the incisal tooth; and (3) the jaw rotation around the hinge axis [5].
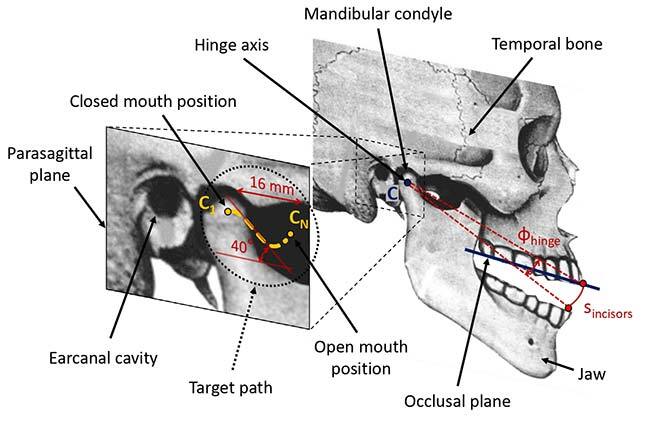
Figure 1: Biomechanical model of the TMJ showing the three parameters defined by the hinge axis (point C): condylar path (C1 CN), incisal path (s_incisors) and jaw rotation (Φ_hinge). [6]
Design of a Six-Bar Mechanism
The designed mechanism shown in Fig.2 makes it possible to reproduce the condylar path with an accuracy of 98.35%, while ensuring the incisal path and jaw rotation around the hinge axis. The originality of our solution does not lie in the mechanism’s architecture as such, but rather in the design method. We separated our mechanism into two sub-mechanisms.
First, an optimization algorithm was used to determine the value of the 11 variables describing the four-bar mechanism formed by l1, l2, l4 and l3 as a part of the triangular member. Using an iterative optimization approach, this algorithm minimized the difference between the path generated at point C and the target condylar path, while ensuring that point A reproduced the incisal path.
Once the two generated paths were validated, we integrated an additional sub-mechanism composed of bars l5 and l6. Thanks to an analytical method, it was possible to constrain the movement of bar l6 so that its rotation corresponds to the one performed by the jaw around the hinge axis, without disrupting the kinematics of the first sub-mechanism.
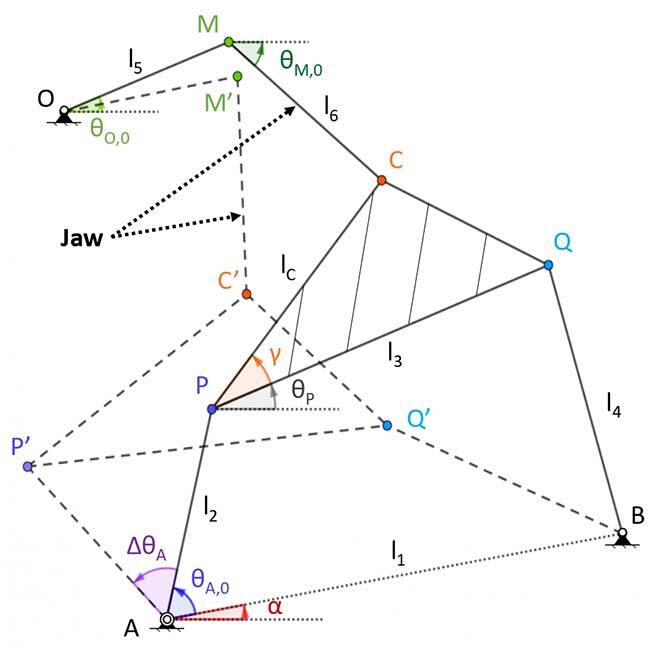
Figure 2: Schematics of the six-bar mechanism with the design variables l1, l2 ,l3, l4, lC, α, θA0, ΔθA, γ, xA, yA for the four-bar sub-mechanism and l5, l6, θO0, θM0, xO, yO for the two-bar sub-mechanism. The initial position is represented as a solid line. [6]

Figure 3: Digital model of the optimized six-bar mechanism. Left: 3D view of the jaw profile with an artificial earcanal; Right: magnified view of the optimized path (dotted line) and the target path (solid line) with the jaw’s orientation along the path generated by the mechanism [6].
Research Opportunities
Coupled with an artificial ear, the kinematic mechanism will provide a test platform allowing a better understanding of the interactions between the jaw and the earcanal. It will then be possible to develop numerical simulations modeling this interaction and to compare numerical and experimental results. The final objective of this project will be to locate and quantify the maximum earcanal deformation and to deduce the associated energy potential. It would then be possible to use an earplug equipped with a piezoelectric energy harvester to capture this energy.
Although the prototype reproduces only one anatomy, the bars of our prototype could be replaced with adjustable bars. Moreover, the triangle could be replaced with a compass with adjustable angles. This would make it possible to simulate several anatomies and consequently several TMJ kinematics. An adjustable mechanism could be used to better understand TMJ disorders and improve the design of mandibular implants.
From a theoretical point of view, the optimization method proposed in our study could help optimize the architecture of biomedical mechanisms with complex kinematics, such as knee or shoulder prostheses.
Additional Information
For more information, please refer to the following research publication:
Demuynck, M., Delnavaz, A., and Voix, J. (June 1, 2021). “Human Temporomandibular Joint Motion: A Synthesis Approach for Designing a Six-Bar Kinematic Simulator.” ASME. J. Mechanisms Robotics. December 2021; 13(6): 065001. https://doi.org/10.1115/1.4050828.